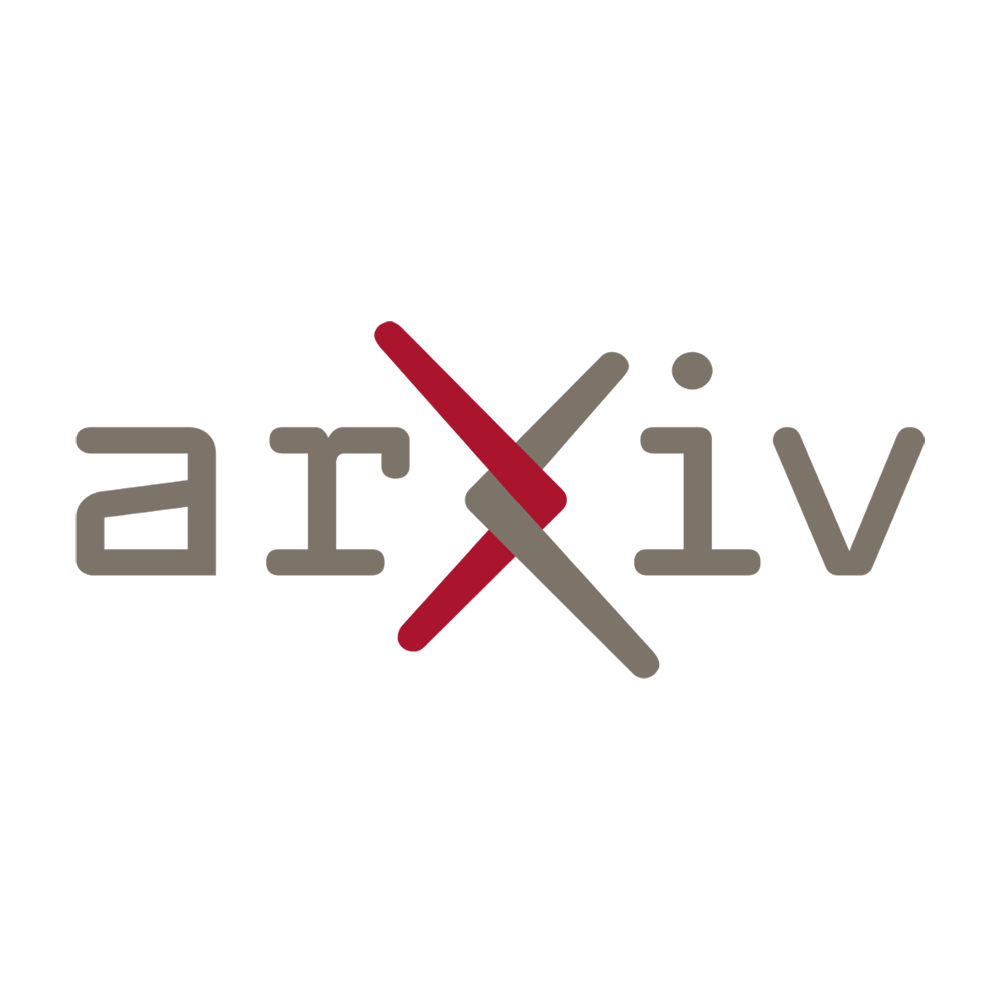
Advances in computational biology have revolutionized our understanding of Gene Regulatory Networks (GRNs), complex systems that govern gene expression and regulation in response to environmental and developmental cues. This article delves into the intricate workings of GRNs, exploring how computational models and algorithms have allowed researchers to unravel their complexities and uncover key insights into the fundamental mechanisms of gene regulation. By studying GRNs, scientists are gaining a deeper understanding of how organisms respond and adapt to their surroundings, offering potential applications in fields such as medicine, agriculture, and synthetic biology. Join us on this journey as we explore the fascinating world of GRNs and the transformative impact of computational biology on our understanding of gene regulation.
Unveiling the Hidden Potential of Gene Regulatory Networks
Gene Regulatory Networks (GRNs) are intricate biological systems that control gene expression and regulation in response to environmental and developmental cues. Advances in computational biology now allow us to delve into the complexity of GRNs and unlock their hidden potential. By exploring the underlying themes and concepts of GRNs in a new light, we can propose innovative solutions and ideas that could revolutionize our understanding of gene regulation.
Understanding Complexity through Network Analysis
GRNs are composed of a vast web of interconnected genes, proteins, and regulatory elements. This unprecedented complexity presents a challenge in unraveling the intricacies of gene regulation. However, by applying network analysis techniques borrowed from fields such as computer science and mathematics, we can gain valuable insights into the behavior of GRNs.
One approach involves visualizing GRNs as complex networks, where nodes represent genes or regulatory elements, and edges represent interactions between them. By studying the properties of these networks, such as node degree and centrality measures, we can identify key regulators and predict their impact on the overall network dynamics. This knowledge can lead to the development of targeted therapies and interventions against diseases that arise from dysregulated gene expression.
Mining GRNs for Therapeutic Targets
Traditionally, drug discovery has focused on targeting individual genes or proteins involved in disease processes. However, by comprehensively understanding GRNs, we can identify network motifs and feedback loops that drive disease progression. These motifs represent potential vulnerabilities that can be exploited for therapeutic intervention.
For example, by identifying genes that act as central hubs in disease-associated GRNs, we can develop drugs that selectively disrupt their interactions and restore normal gene regulation. Additionally, targeting specific regulatory elements, such as microRNAs or transcription factors, can modulate the activity of entire GRNs and restore cellular homeostasis. The exploration of GRNs as therapeutic targets opens up new avenues in precision medicine, leading to more effective and personalized treatments.
Unlocking the Potential of Gene Editing Technologies
Gene editing technologies, such as CRISPR-Cas9, have revolutionized our ability to manipulate DNA sequences with unparalleled precision. Integrating our knowledge of GRNs with gene editing techniques holds great promise for advancing fields like genetic engineering and regenerative medicine.
By understanding how changes in the regulatory elements of GRNs can lead to specific phenotypic outcomes, we can design gene editing strategies that selectively modify these elements to achieve desired changes in cellular behavior. For example, by introducing specific regulatory elements into stem cells, we can guide their differentiation into specific cell types, opening up possibilities for personalized regenerative medicine approaches.
A New Era in Gene Regulation Research
“By exploring the hidden potential of Gene Regulatory Networks, we unlock new possibilities in understanding, treating, and engineering life itself.” – Dr. Lisa Johnson
As computational techniques continue to evolve and our understanding of GRNs deepens, we enter an exciting new era in gene regulation research. The exploration of GRNs can pave the way for personalized medicine, targeted therapies, and groundbreaking advancements in genetic engineering. By leveraging the hidden potential of GRNs, we inch closer to unlocking the mysteries of life itself.
particularly in the field of systems biology, have greatly contributed to our understanding of GRNs. By developing mathematical models and utilizing high-throughput data, researchers have been able to unravel the complexity of these networks and gain insights into their dynamics and functions.
One key aspect of GRNs is their ability to respond to environmental cues. Environmental factors such as temperature, nutrient availability, and stress can trigger specific genes to be turned on or off, leading to appropriate physiological responses. Computational models have allowed us to simulate and predict how GRNs respond to different environmental conditions, providing valuable insights into the underlying mechanisms of gene regulation.
Furthermore, GRNs play a crucial role in development. During embryogenesis, for example, GRNs orchestrate the precise spatial and temporal expression of genes that guide the formation of different tissues and organs. By studying the dynamics of GRNs during development, researchers have been able to identify key regulatory genes and unravel the intricate networks that govern cellular differentiation.
However, despite the significant progress made in understanding GRNs, there are still many challenges and open questions. One major challenge is the sheer complexity of these networks. GRNs can consist of hundreds or even thousands of genes, each with multiple interactions and feedback loops. Analyzing and modeling such complex systems requires powerful computational tools and algorithms.
Another challenge is the integration of different types of data to construct accurate models of GRNs. High-throughput technologies, such as next-generation sequencing and single-cell transcriptomics, generate vast amounts of data on gene expression and protein interactions. Integrating these diverse datasets and extracting meaningful information is a major hurdle that researchers are actively working on.
Looking ahead, the future of GRN research lies in the integration of computational modeling, experimental validation, and new technologies. Advances in machine learning and artificial intelligence will likely play a crucial role in deciphering the intricate dynamics of GRNs. Furthermore, emerging techniques such as genome editing using CRISPR-Cas9 offer exciting possibilities for perturbing GRNs and studying their responses in a controlled manner.
Ultimately, a deeper understanding of GRNs could have far-reaching implications in various fields, including medicine, agriculture, and synthetic biology. By deciphering the regulatory mechanisms that control gene expression, we may be able to develop targeted therapies for genetic diseases, engineer crops with improved traits, and design synthetic gene circuits for biotechnological applications.
In conclusion, the study of GRNs has come a long way thanks to advancements in computational biology. As we continue to explore and unravel the complexity of these networks, we can expect new insights and breakthroughs that will further our understanding of gene regulation and its role in various biological processes.
Read the original article