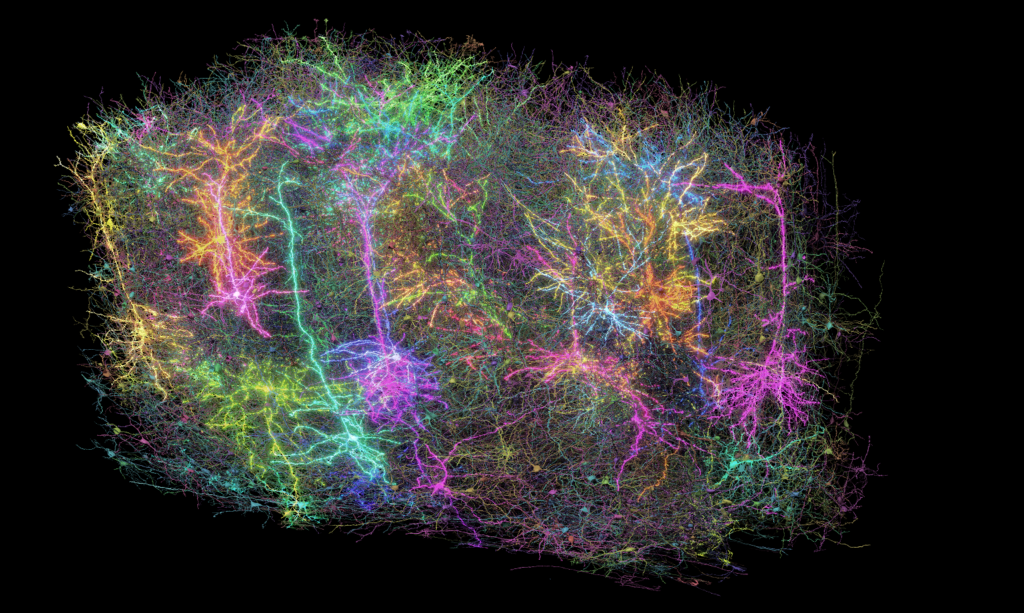
A map of nerve cell connectivity, form, and function from within a grain-of-sand-sized portion of the brain is published today, marking not just a scientific marvel but a step towards the ‘impossible’ goal of understanding the elusive origins of thought, emotion, and consciousness.
Frances Crick, who took up neuroscience after sharing the Nobel prize for co-discovering DNA’s double helix, wrote in 1979 about what he believed were the dismal prospects for understanding the detailed workings of the brain: ‘It is no use asking for the impossible, such as, say, the exact wiring diagram for a cubic millimetre of brain tissue and the way all its neurons are firing.’
But, after seven years of toil, a worldwide team of more than 150 researchers has come close to achieving Crick’s impossible feat. Today they unveil a detailed map of one cubic millimetre of mouse brain.
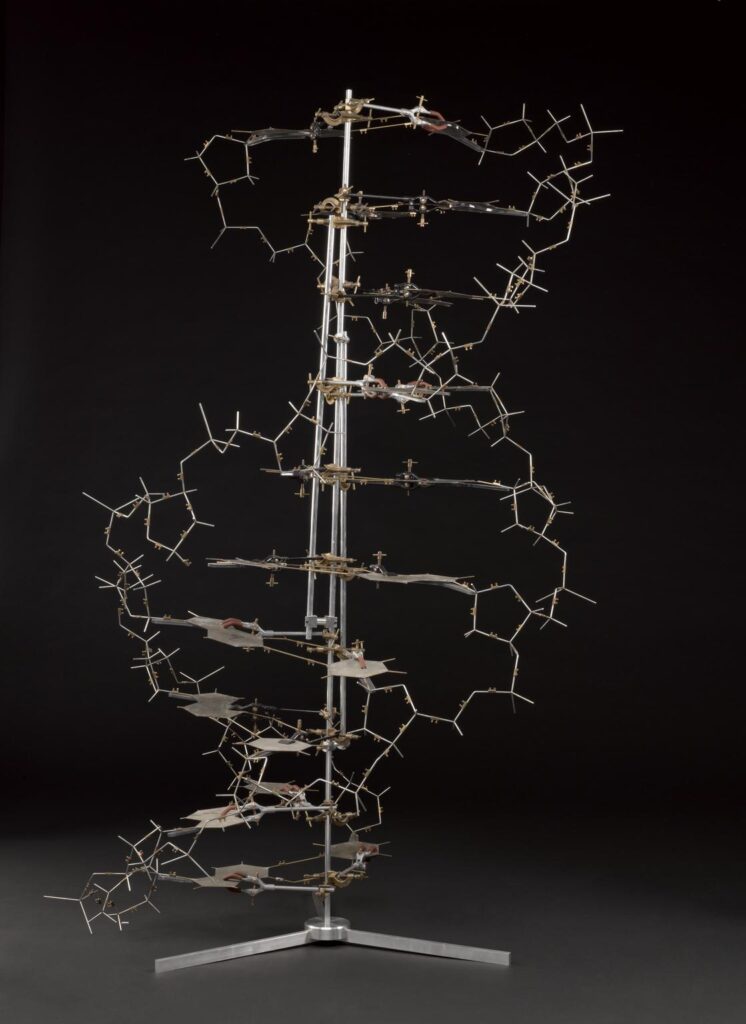
The project relied on using mice that have been genetically modified with a protein that makes their neurons fluoresce when they are active, so the neuroscientists could not only trace the wiring diagrams of a tiny part of the mouse brain but show how its circuits responded to stimuli.
Scientists at Baylor College of Medicine in Houston, Texas, began by using microscopes to record the brain activity within the one cubic millimetre portion of the visual cortex as the animal watched various movies and YouTube clips.
Afterwards, Allen Institute researchers took that same cubic millimetre of the brain and sliced it into more than 25,000 layers, each 1/400th the width of a human hair, and took high-resolution electron microscopy pictures of each slice. Finally, another team at Princeton University used artificial intelligence and machine learning to reconstruct how the cells and connections were arranged in three dimensions.
The result is the biggest wiring diagram of its kind, what one researcher called ‘an exquisite forest,’ containing more than 200,000 cells, an estimated four kilometres of axons (the branches that reach out to other cells) and 523 million synapses (the connection points between cells) and, when combined with the recordings of brain activity, it can also reveal the neurons at work as they process visual information.
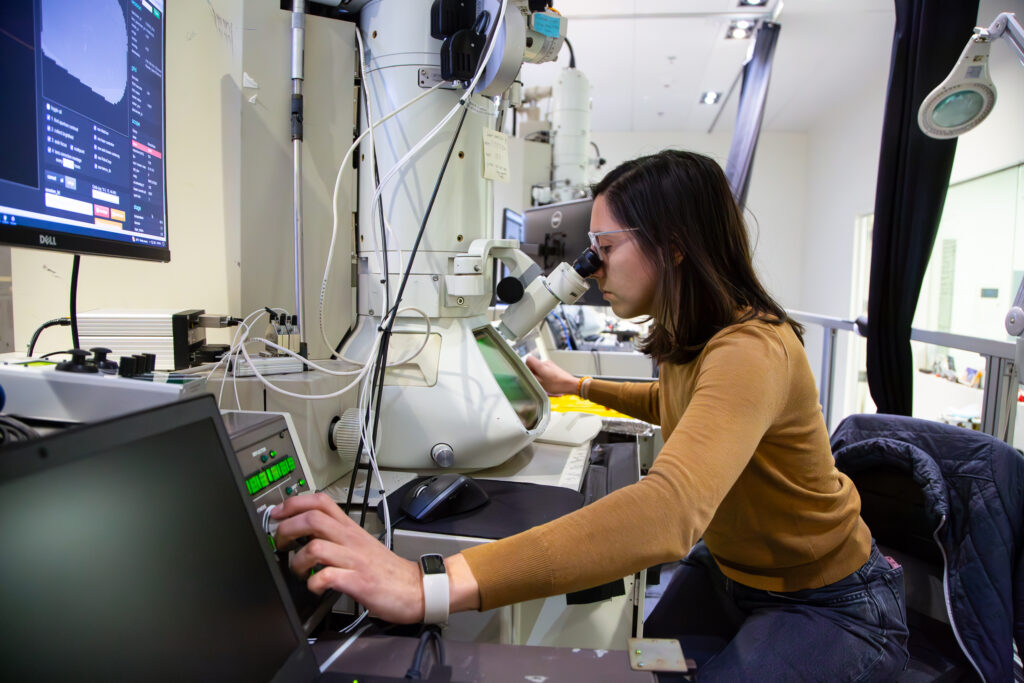
Andreas Tolias, who worked on this project at both Baylor College of Medicine and Stanford University, used functional data measuring the activity of neurons in the visual cortex to build what is called a ‘foundation model’, where an AI could be trained—for instance, using Mad Max clips. This foundation model was then used to create a ‘digital twin’ to predict how a particular neuron would respond when shown stimuli it had not been trained on, such as novel videos, static images, moving dots, and so on.
‘A foundation model is built from many experiments across multiple mice,’ said Tolias. ‘We can then use this model to create digital twins of individual mice and neurons.’
The team found that when neurons responded reliably to visual stimuli, the twin was able to predict their reactions even to entirely new kinds of stimulus – demonstrating the power of this approach to build accurate functional models of the brain. Building brain foundation models and digital twins on the behaviour of actual mouse brain tissue opens the possibility of directly comparing biological and artificial intelligence – comparisons that could prove valuable for advancing both fields.
The mouse brain mapping project, which is called MICrONS (Machine Intelligence from Cortical Networks), is described in ten studies published today in the Nature family of journals, along with 1.6 petabytes of data (equivalent to 22 years of non-stop HD videos). The efforts mark ‘a watershed moment for neuroscience, comparable to the Human Genome Project in their transformative potential,’ says David Markowitz, who coordinated this work.
One surprising finding by Casey Schneider-Mizell of the Allen and colleagues was the discovery of a more sophisticated kind of inhibition within the brain. Scientists previously thought of inhibitory cells as suppressing the activity of nearby nerve cells, but the study showed they can be highly selective about which cells they target.
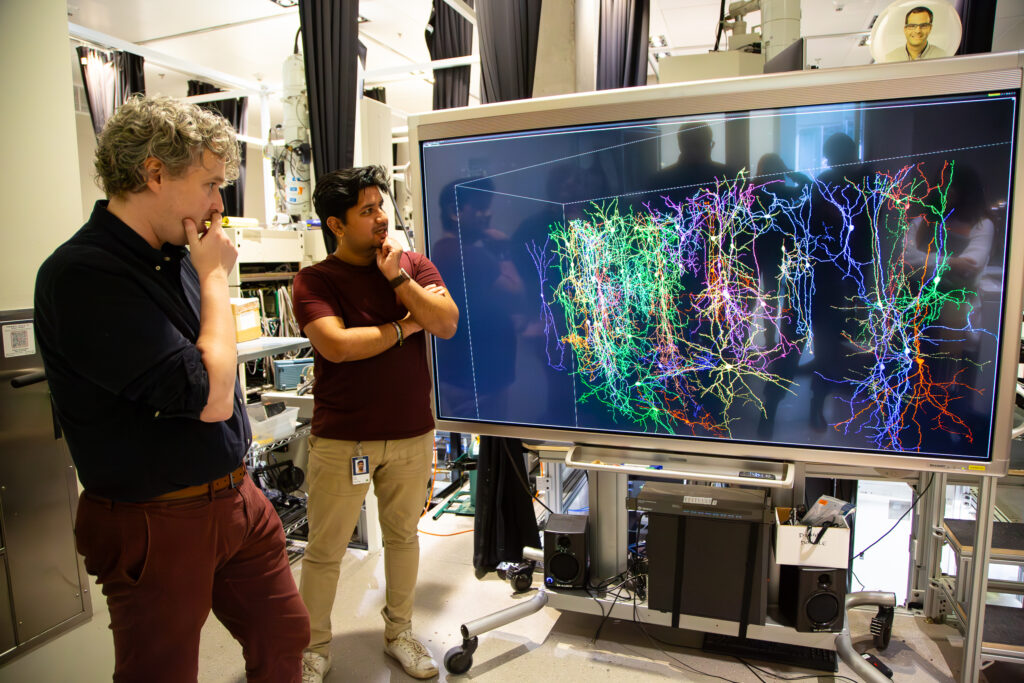
Understanding the brain’s form and function down to the level of individual brain cells has implications for understanding disorders like Alzheimer’s, Parkinson’s, autism, and schizophrenia that involve disruptions in neural communication. Nuno da Costa of the Allen Institute says: ‘We are describing a kind of Google map or blueprint of this grain of sand. In the future, we can use this to compare the brain wiring in a healthy mouse to the brain wiring in a model of disease.’
He adds that, when it comes to extending the work, the US National Institutes of Health, NIH, is funding several efforts to show the feasibility over the next four years, before tackling the entire mouse brain. ‘We could already show you a section of a hemisphere of a mouse (centimetre size) imaged at the same resolution as the millimetre scale MICrONS dataset,’ he says.
For the human brain, the NIH supports a related programme with a more modest objective of mapping single cells and their projections.
Forrest Collman of the Allen comments that the human brain is about 1,300 times larger than a mouse brain. ‘There are even larger technical, economic and ethical problems with doing a whole human brain in this way, and most scientists do not think this is something practical to pursue any time soon,’ he says. ‘On the other hand,’ he adds, ‘small pieces of human brain can be examined with this method and certain aspects can be compared in order to understand how general the features are we are learning about mouse brains.’
The current generation of artificial intelligence, which is trained on data to learn, is very loosely modelled on the connectivity of the brain. But, says Collman, many aspects of biology are absent from contemporary AI models: ‘This includes the vast diversity of cell types and the myriad rules and principles that shape the plasticity of synapses in our brains.’
Today’s detailed view of the mouse brain provides a glimpse of the Byzantine complexity of the cell types and their connections, which might inspire new kinds of AI. But he adds: ‘Clearly though, at some level, we still have a lot to learn about how natural intelligence functions and learns so efficiently, quickly and with so little data compared to present day AI systems.’
The post The most complete map of a brain is unveiled today appeared first on Science Museum Blog.