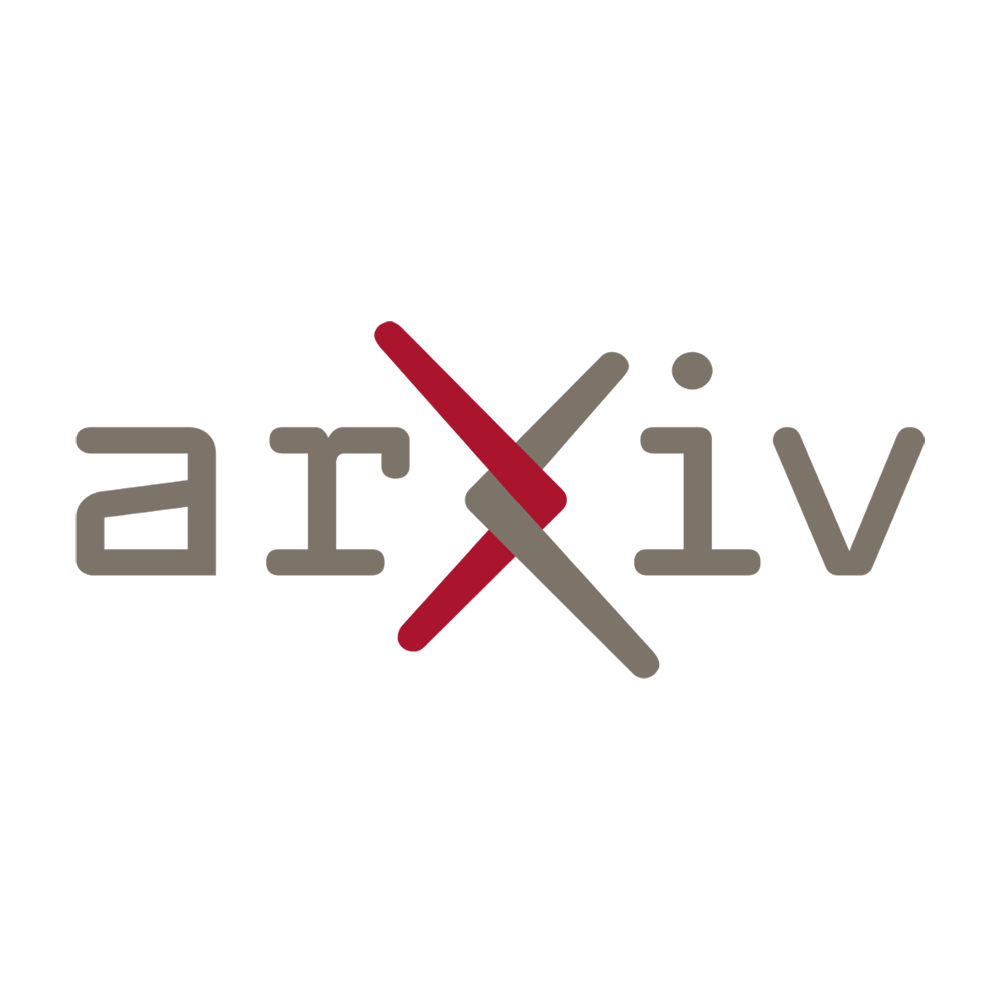
The Imitation Game for Educational AI
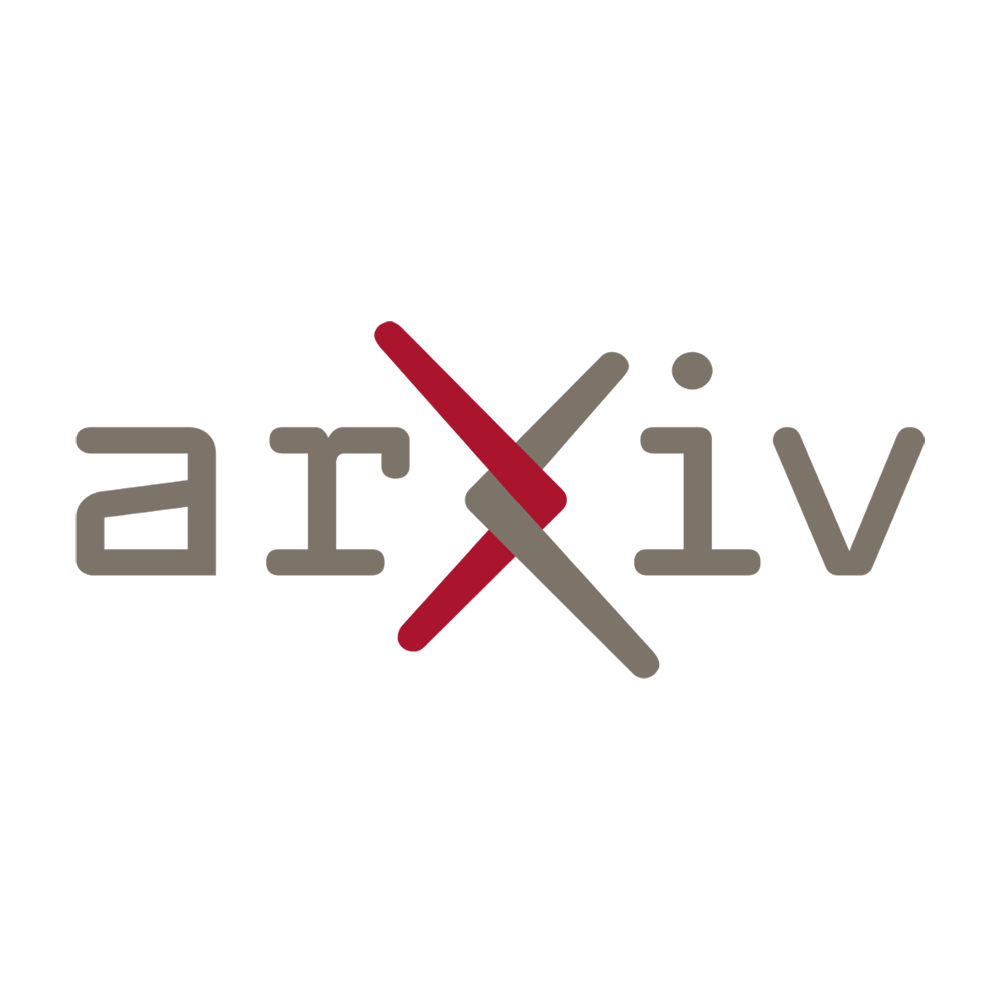
arXiv:2502.14184v1 Announce Type: new Abstract: Understanding the relationship between the evolution of microstructures of irradiated LiAlO2 pellets and tritium diffusion, retention and release could improve predictions of tritium-producing burnable absorber rod performance. Given expert-labeled segmented images of irradiated and unirradiated pellets, we trained Deep Convolutional Neural Networks to segment images into defect, grain, and boundary classes. Qualitative microstructural information was calculated from these segmented images to facilitate the comparison of unirradiated and irradiated pellets. We tested modifications to improve the sensitivity of the model, including incorporating meta-data into the model and utilizing uncertainty quantification. The predicted segmentation was similar to the expert-labeled segmentation for most methods of microstructural qualification, including pixel proportion, defect area, and defect density. Overall, the high performance metrics for the best models for both irradiated and unirradiated images shows that utilizing neural network models is a viable alternative to expert-labeled images.
Unraveling the Potential of Quantum Computing: A Revolutionary Leap in Computing Technology
In the ever-evolving world of technology, quantum computing has emerged as a groundbreaking concept that promises to revolutionize the way we process information. With its ability to solve complex problems at an unprecedented speed, quantum computing has the potential to transform various industries, from finance and healthcare to cybersecurity and logistics.
To understand the potential of quantum computing, it is essential to first grasp the fundamental principles that differentiate it from classical computing. While classical computers use bits to represent information as either a 0 or a 1, quantum computers utilize quantum bits, or qubits, which can exist in multiple states simultaneously. This phenomenon, known as superposition, allows quantum computers to perform calculations exponentially faster than their classical counterparts.
One of the most significant advantages of quantum computing lies in its ability to solve problems that are currently intractable for classical computers. For instance, quantum computers can efficiently factor large numbers, a task that would take classical computers an impractical amount of time. This capability has significant implications for cryptography, as it renders many encryption methods vulnerable to quantum attacks. Quantum computing also has the potential to revolutionize drug discovery by simulating molecular interactions and accelerating the identification of potential drug candidates.
Another area where quantum computing can make a substantial impact is optimization. Many real-world problems, such as route optimization, portfolio management, and supply chain optimization, involve finding the best solution among a vast number of possibilities. Classical computers struggle to handle these problems efficiently, but quantum computers can explore all possible solutions simultaneously, providing optimal results in a fraction of the time.
Furthermore, quantum computing can enhance machine learning algorithms, leading to more accurate predictions and insights. By leveraging its ability to process vast amounts of data simultaneously, quantum computers can identify patterns and correlations that would be challenging for classical computers to detect. This could have profound implications for fields like artificial intelligence, where the ability to process and analyze large datasets is crucial.
Despite its immense potential, quantum computing is still in its infancy. Building a practical quantum computer is an incredibly complex task that requires overcoming numerous technical challenges, such as maintaining the stability of qubits and minimizing errors caused by environmental factors. However, significant progress has been made in recent years, with companies like IBM, Google, and Microsoft actively developing quantum computers and exploring their applications.
As quantum computing continues to advance, it is crucial to address the potential risks associated with this powerful technology. Quantum computers have the potential to break many of the encryption methods currently used to secure sensitive information. Therefore, it is essential to develop quantum-resistant encryption algorithms to ensure the security of data in the post-quantum era.
In conclusion, quantum computing represents a revolutionary leap in computing technology, with the potential to transform various industries and solve problems that are currently unsolvable. While there are still many challenges to overcome, the progress made in recent years indicates that quantum computing is no longer just a theoretical concept but a tangible reality. As researchers and scientists continue to unravel its potential, the future of quantum computing holds immense promise for a faster, more efficient, and more secure world.
arXiv:2502.13194v1 Announce Type: cross Abstract: In this paper we present a novel approach for multiagent decision making in dynamic environments based on Factor Graphs and the Max-Sum algorithm, considering asynchronous variable reassignments and distributed message-passing among agents. Motivated by the challenging domain of lane-free traffic where automated vehicles can communicate and coordinate as agents, we propose a more realistic communication framework for Factor Graph formulations that satisfies the above-mentioned restrictions, along with Conditional Max-Sum: an extension of Max-Sum with a revised message-passing process that is better suited for asynchronous settings. The overall application in lane-free traffic can be viewed as a hybrid system where the Factor Graph formulation undertakes the strategic decision making of vehicles, that of desired lateral alignment in a coordinated manner; and acts on top of a rule-based method we devise that provides a structured representation of the lane-free environment for the factors, while also handling the underlying control of vehicles regarding core operations and safety. Our experimental evaluation showcases the capabilities of the proposed framework in problems with intense coordination needs when compared to a domain-specific baseline without communication, and an increased adeptness of Conditional Max-Sum with respect to the standard algorithm.
Exploring the Vast Universe: Unveiling the Mysteries of Cosmology
The universe has always been a subject of fascination for humanity. Its vastness, complexity, and the mysteries it holds have captivated scientists, philosophers, and ordinary people alike. Cosmology, the study of the universe as a whole, aims to unravel these mysteries and understand the fundamental nature of our existence. Through centuries of observation, experimentation, and theoretical advancements, cosmologists have made significant progress in uncovering the secrets of the cosmos.
One of the most profound questions in cosmology is the origin of the universe itself. The prevailing theory, known as the Big Bang theory, suggests that the universe began as an incredibly hot and dense singularity around 13.8 billion years ago. This singularity then rapidly expanded, giving rise to the vast expanse of space and time we observe today. However, the exact mechanisms that triggered the Big Bang and what happened in the first moments of the universe remain elusive.
To understand the evolution of the universe, cosmologists rely on a variety of tools and techniques. Observational astronomy plays a crucial role in gathering data about distant galaxies, stars, and other celestial objects. Telescopes, both on the ground and in space, allow scientists to peer into the depths of the universe, capturing images and spectra that provide valuable insights into its composition, structure, and history.
In recent years, technological advancements have revolutionized our ability to explore the cosmos. The Hubble Space Telescope, for example, has provided breathtaking images of distant galaxies, revealing their intricate shapes and colors. Satellites like the Planck mission have mapped the cosmic microwave background radiation, the faint afterglow of the Big Bang, providing vital clues about the early universe’s conditions.
Cosmologists also rely on theoretical models and simulations to understand the universe’s behavior on a grand scale. These models incorporate our current understanding of fundamental physics, such as Einstein’s theory of general relativity, to describe the dynamics of space, time, and matter. By running simulations on powerful supercomputers, scientists can recreate the evolution of the universe from its early stages to the present day, testing different scenarios and comparing the results with observational data.
One of the most intriguing aspects of cosmology is the nature of dark matter and dark energy. These two mysterious entities, which cannot be directly observed, are believed to make up the majority of the universe’s mass and energy. Dark matter, although invisible, exerts a gravitational pull on visible matter, shaping the large-scale structure of the cosmos. Dark energy, on the other hand, is thought to be responsible for the accelerated expansion of the universe. Understanding the properties and origins of dark matter and dark energy is a major focus of current cosmological research.
Cosmology also addresses the question of whether we are alone in the universe. The discovery of exoplanets, planets orbiting stars outside our solar system, has fueled the search for extraterrestrial life. By studying the conditions necessary for life on Earth and exploring the potential habitability of exoplanets, scientists hope to find clues about the existence of life elsewhere in the universe.
As our knowledge of the universe expands, so do the questions we ask. Cosmology is a field that constantly pushes the boundaries of human understanding, challenging our preconceived notions and inspiring new avenues of research. By exploring the vast universe and unveiling its mysteries, cosmologists not only satisfy our innate curiosity but also contribute to our collective knowledge of the cosmos and our place within it.
Unveiling the Enigmatic Singularities of Black Holes
Black holes have long captivated the imagination of scientists and the general public alike. These enigmatic cosmic entities, with their immense gravitational pull, have been the subject of countless studies and research. While much has been learned about black holes over the years, one aspect that continues to baffle scientists is the existence of singularities within them.
A singularity is a point in space-time where the laws of physics break down. It is a region of infinite density and zero volume, where matter is crushed to an unimaginable extent. In the case of black holes, singularities are believed to exist at their centers, hidden behind the event horizon – the point of no return beyond which nothing, not even light, can escape.
The concept of a singularity was first proposed by physicist Albert Einstein in his theory of general relativity. According to this theory, when a massive star collapses under its own gravitational force, it forms a singularity. The collapse is so intense that it creates a gravitational well from which nothing can escape, giving rise to a black hole.
However, the existence of singularities poses a fundamental problem for physicists. The laws of physics, as we currently understand them, cannot describe what happens within a singularity. At such extreme conditions, both general relativity and quantum mechanics, the two pillars of modern physics, fail to provide a coherent explanation.
This discrepancy between general relativity and quantum mechanics is known as the “black hole information paradox.” According to quantum mechanics, information cannot be destroyed, and yet, if a singularity exists within a black hole, all the information contained in the matter that fell into it would be lost forever.
Several theories have been proposed to resolve this paradox and shed light on the nature of singularities. One such theory is loop quantum gravity, which attempts to reconcile general relativity with quantum mechanics. According to this theory, space-time is not continuous but rather composed of discrete units or “quanta.” By applying the principles of quantum mechanics to space-time itself, loop quantum gravity suggests that singularities may not be infinitely dense but rather replaced by a “quantum bounce,” preventing the formation of a true singularity.
Another theory, known as string theory, proposes that the fundamental building blocks of the universe are not particles but tiny vibrating strings. In the context of black holes, string theory suggests that the singularity is not a point but rather a “brane,” a higher-dimensional object. This theory opens up the possibility of a wormhole connecting the singularity to another region of space-time, allowing information to escape.
While these theories offer intriguing possibilities, they are still purely speculative, and experimental evidence to support them is lacking. The extreme conditions within black holes make it nearly impossible to directly observe or study singularities. However, advancements in observational techniques, such as gravitational wave detectors, may provide valuable insights into the nature of black holes and their singularities in the future.
In conclusion, the enigmatic singularities of black holes continue to challenge our understanding of the laws of physics. These regions of infinite density and zero volume lie at the heart of black holes, defying our current theories. Resolving the black hole information paradox and unraveling the mysteries of singularities remains one of the most significant challenges in modern physics. Only through continued research and exploration will we be able to lift the veil on these cosmic enigmas and gain a deeper understanding of the nature of our universe.